APPLICATION GUIDE
When it comes to choosing the right permanent magnetic material, several key factors should be carefully considered. Each decision plays a crucial role in determining the effectiveness of the magnet for a particular application. Let’s explore these important characteristics in a more conversational tone:
Flux Requirement: Think of it like this – every application has its own magnetic needs. Understanding the flux requirement ensures that the chosen magnetic material aligns perfectly with the job at hand.
Maximum Operating Temperature: Just like how we can’t handle extreme temperatures, magnets have their limits too. Knowing the maximum operating temperature is like giving the magnet a comfort zone to perform at its best without breaking a sweat.
Cost and Availability: Money matters, and so does accessibility. We consider the cost of the material and its availability because, let’s face it, a solution that breaks the bank and is hard to find isn’t really a solution.
Corrosion Resistance: Nobody likes rust, not even magnets. Predicting the level of corrosion a magnet might encounter helps us choose a material that stands strong against the elements.
Magnetic Stability: Think of it as the magnet’s personality – some magnets are steadfast and reliable, while others may be a bit more unpredictable. Knowing the level of magnetic stability required ensures we pick the right magnet for the job.
Size and Weight Constraints: Imagine trying to fit into your favorite jeans – magnets have size and weight limitations too. Understanding these constraints helps us choose a material that fits snugly into the available space.
Now, let’s talk about the big players – Alnico, Ferrite, Samarium Cobalt (SmCo), and Neodymium-Iron-Boron (NdFeB). These are the A-listers in the magnetic world, each with its unique strengths. It’s like choosing between rock, pop, jazz, and hip-hop – they all have their fan base.
Oh, and there’s this interesting tidbit: some magnets are a bit picky about their magnetization direction – it’s like they have their own favorite pose. Others are more flexible and can magnetize in any direction. It’s a bit like choosing between a strict dance routine and freestyle – both have their charm.
So, when delving into the world of permanent magnetic materials, think of it as assembling your dream team – each member chosen for their unique strengths and abilities.
Comparing the magnetic performance of various types and grades of permanent magnets is most conveniently done by evaluating their maximum energy product (BHmax). This metric signifies the point at which a magnet delivers the highest energy output relative to its minimum volume.
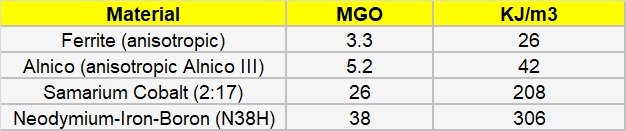
Magnetic energy product (MGO) in CGS units corresponds to kilojoules per cubic meter (kJ/m³) in the SI unit system.
It is common to require information about the flux density on the pole face of a magnet. Frequently, there is a misconception that this value is equivalent to Remanence (Br). However, Remanence only represents induction in closed circuit conditions and does not accurately reflect the actual flux density in typical working scenarios.
The table below illustrates typical pole face flux densities for the four grades when operating at or near their BHmax points.

The impact of temperature is the primary factor influencing magnet stability. However, exposure to high external magnetic fields can also affect certain types of magnets, and the degree of this effect varies.

TIME
The impact of time on magnets is minimal, averaging a loss of less than 1 x 10-5 per annum at 20 ᵒC. Over a 100,000-hour period (approximately 11.4 years), these losses vary from practically zero for SmCo to less than 3% for Alcomax III at low permeance coefficients.
SHOCK & VIBRATION
For modern magnet materials, shock and vibration are generally not problematic, except for the most precisely calibrated devices. However, some magnet materials, particularly SmCo, may be brittle and susceptible to fracture from mechanical impact.
RADIATION
In particle beam deflection applications, magnets with higher Hci are recommended. Tests indicate that SmCo experiences significant losses when exposed to high radiation levels (109 to 1010 rads), while NdFeB loses 50% at 4 x 106 rads and 100% at 7 x107 rads. At low radiation levels, losses align with those attributed to temperature changes. It’s worth noting that certain magnet materials contain Cobalt, which can retain radiation after exposure.
SHAPE
The shape of a magnet influences its performance and stability. The magnet’s shape determines its position along the demagnetization curve. The higher up the curve a magnet operates, the closer it is to its optimal working point, making it less susceptible to external influences. Magnets used in closed magnetic circuits or with longer lengths tend to perform better and exhibit greater magnetic stability.
Enhancing magnetic stability can be achieved by exposing the magnet in advance to potential detrimental influences through techniques such as thermal cycling and controlled demagnetizing fields (ageing).
Another factor contributing to performance loss is the breakdown of the composition due to environmental effects, leading to structural issues such as corrosion or, in the case of NdFeB, exposure to hydrogen.
The primary factor affecting magnet stability is temperature, but exposure to high external magnetic fields can also influence specific types of magnets to varying degrees.

TIME
The impact of time on magnets is negligible, resulting in an average loss of less than 1 x 10-5 per annum at 20 ᵒC. Over a 100,000-hour period (equivalent to 11.4 years), these losses range from practically zero for SmCo to less than 3% for Alcomax III at low permeance coefficients.
SHOCK & VIBRATION
Historically, shock and vibration posed challenges for magnets, but with modern magnet materials, this is generally no longer a concern, except for highly calibrated devices. However, some magnet materials, particularly SmCo, may be brittle and susceptible to mechanical impact-induced fractures.
RADIATION
In particle beam deflection applications, it is advisable to use magnets with higher Hci. Tests indicate that SmCo experiences substantial losses when exposed to elevated radiation levels (109 to 1010 rads), while NdFeB experiences a 50% loss at 4 x 106 rads and a complete loss at 7 x 107 rads. At lower radiation levels, losses align with those associated with temperature changes. Notably, certain magnet materials containing Cobalt can retain radiation after exposure.
SHAPE
Magnet shape does influence performance and, consequently, stability. The magnet’s shape determines its position along the demagnetization curve. Operating higher up the curve brings the magnet closer to its optimal working point, making it less vulnerable to external influences. Magnets used in closed magnetic circuits or with longer lengths generally exhibit better performance and greater magnetic stability.
Enhancing magnetic stability can be achieved by pre-exposing the magnet to potential detrimental influences through techniques such as thermal cycling and controlled demagnetizing fields (ageing).
Another factor contributing to performance loss is the breakdown of the composition due to environmental effects, leading to structural issues such as corrosion, or, in the case of NdFeB, exposure to hydrogen.
The pricing of individual magnets is influenced by factors such as shape, tolerances, and quantity, but the most substantial impact comes from the cost of the fundamental raw material.

Indeed, tooling is often necessary when introducing new sizes or engaging in volume production. Similarly, fixtures may be required for precision machining in situations where close tolerances need to be maintained.
Path of Least Resistance: Flux lines follow the paths of least resistance, or in magnetic terms, the path of greatest permanence (lowest reluctance).
Repulsion of Flux Lines: Flux lines repel each other if their direction of flow is the same, and they can never cross.
Shortest Path: Flux lines always follow the shortest path through any medium.
Curved Paths: Flux lines typically move in curved paths.
Right Angles Entry/Exit: Flux lines always leave and enter the surfaces of ferromagnetic materials at right angles.
Saturated Ferromagnetic Materials: Ferromagnetic materials have a limited ability to carry flux. When saturated, they behave as if they were not there, similar to an air gap.
North to South Pole Flow: Flux lines always travel from the nearest north pole to the nearest south pole, forming a closed loop path.
There are two distinct categories of magnetization changes: reversible and irreversible. Reversible changes are influenced by temperature and depend on the material composition, unaffected by the magnet’s shape, size, or position on the demagnetization curve. These losses vanish completely when the magnet is returned to its original temperature, without the need for remagnetization. On the other hand, irreversible losses only occur after surpassing a certain temperature threshold. Operating at a higher working point can help limit these losses. Unrecoverable losses occur when excessive temperatures lead to metallurgical changes within the magnet.
REVERSIBLE EFFECT OF TEMPERATURE (20C – 150 C)

MAXIMUM WORKING TEMPERATURES (BEFORE IRREVERSIBLE LOSSES COMMENCE)
The maximum working temperature of a magnet is determined by its working point in the circuit. A higher working point allows the magnet to operate at elevated temperatures.

N.B. Irreversible losses may be restored by remagnetising the magnet
UNRECOVERABLE LOSSES (CURIE TEMPERATURE)
Every material has a specific maximum temperature at which metallurgical changes take place within the magnet structure, leading to the breakdown of individual magnetic domains. Once these losses occur, they cannot be reversed through re magnetization.

EFFECTS OF SUB-ZERO TEMPERATURES
The impact of low temperatures varies across material groups and is closely tied to the magnet’s shape, influencing its working point on the demagnetization curve.

Greater strength does not always translate to better performance; magnetic design involves considering multiple factors, with flux strength being just one of them.
Introducing a steel pole piece can enhance a magnet’s effectiveness by redirecting the flux to a more beneficial part of the magnetic circuit.
Always consider the working temperature of your application, as temperature poses the most significant threat to magnetic stability; incorporate it into your design and material/grade selection.
In holding/attracting applications, two poles are preferable, although it’s important to minimize the air gap between the magnet and the object to be attracted, as the flux won’t travel as far.
Focusing flux lines with Rare Earth Magnets can be challenging, and the use of steel poles is generally ineffective in this case.
Replicating the application is the best test for a magnetic device since there is no single, simple test that provides comprehensive information about a magnet; including the magnet’s anticipated work conditions in your testing procedure is often the most effective approach.
Permanent magnets find applications across various industries, and they can be classified into the following sections based on their functions:
Conversion of Electrical Energy to Physical Motion:
- Actuators
- Speakers
- Motors
- Meters
- Other instrumentation
Conversion of Physical Motion into Electrical Energy:
- Generators
- Microphones
- Sensors
Producing Mechanical Energy:
- Holding
- Lifting
- Attracting
- Repelling
- Conveying
- Driving
- Separating
Controlling Fields:
- Annealing
- Plasma control
- Sputtering
- Focusing
- NMR (Nuclear Magnetic Resonance)
Mechanical to Heat:
- Eddy Current and Hysteresis drives